Generic Heat Exchanger Component
Description
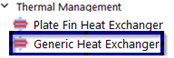
The Generic Heat Exchanger (GHX) requires that the heat exchanger performance is known. There are several options to specify the performance such as: temperature change, thermal duty, NTU, effectiveness, and the Hs parameter.
Generic Heat Exchanger can be used in Compressible and Incompressible (hydraulic and non-hydraulic) simulations. Only a simple phase change is available, and it should be used with caution. The component has four fluid connections, and it models heat exchange between two streams in a network. Heat Exchangers in Flow Simulator come with 4 hidden chambers representing the 4 sides of a heat exchanger. The Generic Heat Exchanger uses Orifice or Effective Area elements (Compressible or Incompressible) in the backend to model restrictions losses (pressure loss) of both flow paths based on user defined loss parameters and characteristic flow area. The Heat addition/removal is calculated based on user inputs for Heat Exchanger Performance curves in Generic Hx module and Q (Heat Addition/Removal) is supplied to Orifice or Effective Area Elements to predict temperatures of the exiting fluids.
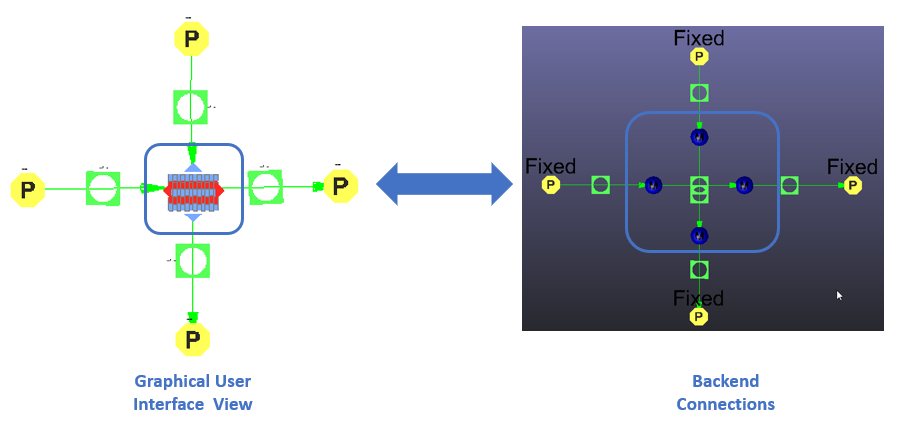
- The Hot Side circuit must get connected to Line 1 (Indicated by Red Color
part of Component Image) and Cold Side circuit get connected to Line 2
(Indicated with Blue Color part of Component Image) as shown in the image below.
Figure 3. - You can choose to model additional inlet and outlet losses using the separate Discrete Loss/Tube Element upstream and downstream of heat exchanger respectively.
- The Hot/Cold Side circuit line must be connected with either compressible or Incompressible set of elements. Mixing of elements sets for a Hot/Cold side circuit line is not allowed. Below Table represents some modeling/allowable scenarios.
Modeling/Allowable Scenarios | Flow Simulator Network |
---|---|
Hot Side: Compressible Gases Cold Side: Compressible Gases Example: Air to Air Heat Exchanger |
![]() |
Hot Side : Incompressible Liquids Cold Side : Incompressible Liquids Example: Fuel Cooled Oil Cooler (FCOC) |
![]() |
Hot Side: Incompressible Liquids Cold Side: Compressible Gases Example: Air Cooled Oil Cooler (ACOC) |
![]() |
Hot Side: Compressible Gases Cold Side: Incompressible Liquids Example: Air to Liquid Heat Exchanger |
![]() |

Flow Simulator can also be used for Heat Exchanger design. For heat exchanger design a detailed representation of the heat exchanger is required. The example below shows flow elements representing the cold and hot side with thermal network resistors connecting the sides. Heat exchanger performance can be predicted from such a model.
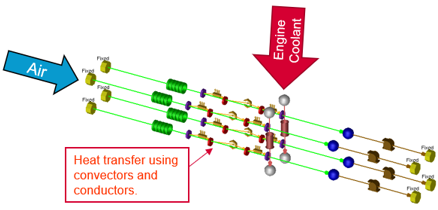
Generic Heat Exchanger Element Inputs
Table of the inputs for the Generic Heat Exchanger Component.
Element Specific Generic Heat Exchanger Component Input Variables | ||
---|---|---|
Index | UI Name (.flo label) | Description |
2,10 | Geometric Input Type (CS_SHAPE_COLD, CS_SHAPE_HOT) | Type of geometry information: 0. Enter Pipe Area only (assume Hydraulic Diameter from assumed circular shape). 1. Enter Pipe Area and Hydraulic Diameter. |
3,11 | Cross Sectional Area (AREA_COLD, AREA_HOT) |
Flow area for hot and cold side fluids. |
4,12 | Hydraulic Diameter (HYD_DIA_COLD, HYD_DIA_HOT) |
Hydraulic Diameter for hot and cold side fluids. |
5,13 | Pressure Loss Options (PLOSS_COLD, PLOSS_HOT) |
Options to specify type of pressure loss modeling: 0. No Loss 1. Fixed Loss Coefficient 2. Fixed Total Pressure Drop 3. Flow vs. Delta.P (PTIN - PSEX) 4. Velocity vs. Delta.P (PTIN - PSEX) 5. Loss Coefficient vs. Reynolds Number 16. Flow vs. Delta P vs. Temperature |
6,14 | Loss Coefficient (KLOSS_COLD, KLOSS_HOT) |
Incompressible Loss Coefficient |
7,15 | Delta Total Pressure (DELTA_PT_COLD, DELTA_PT_HOT) |
Total pressure drop across a flow path. |
8,16 | Mass or Volume (MASS_VOL_COLD, MASS_VOL_HOT) | Type of flow input for PLOSS = 3 and 16 0. Flow in lbm/sec 1. Flow in gallons per minute |
9,17 | Mass or Volume (NPRCD_COLD, NPRCD_HOT) |
Number of values in PLOSS tables |
18-24 | Not used | |
25 | Heat Transfer Options (HOPT) | Options for modeling Heat Transfer between Hot and Cold side
fluids: 1. Thermal Duty: Heat Input 2. Hot Fluid Delta.T 3. Cold Fluid Delta.T 4. Effectiveness 5. Effectiveness vs. Flow_Rate_Cold vs. Flow_Rate_Hot 8. Effectiveness vs. NTU vs. Heat Capacity Ratio 9. Nusselt Number vs. RE_Cold vs. RE_Hot 10. Constant hA coefficient value 12. Fixed Hot Fluid Exit T 13. Fixed Cold Fluid Exit T 14. Hs Constant 15. Hs vs Flow_Rate_Cold vs. Flow_Rate_Hot 16. Hot Fluid Exit Quality 17. Cold Fluid Exit Quality 18. NTU Constant |
26 | Heat Input (QIN) | Heat Addition or Quality at the exit of the hot or cold stream. Quality is the mass fraction of vapor in the fluid. A quality of 0 is all liquid and 1 is all vapor. |
27 | Fluid Delta.T (DELT_T) | Delta Total Temperature for Hot (or Cold) Side fluid |
28 | Effectiveness (EFFECTIVENESS) | Effectiveness of Heat Exchanger |
29 | Type of Configurations (CONFIG_TYPE) |
Heat Exchangers Flow Configurations 0. Parallel Flow 1. Counter Flow 2. Shell and Tube 3. Cross flow both flows unmixed Cross flow One fluid mixed |
30 | Number of Shell Passes (NUM_SHELL_PASS) | No. of Shell Passes for Shell and Tube Hx Configurations |
31 | Primary hA coefficient (PRI_HA) | Htc*Area Coefficient of Primary side fluid |
32 | Secondary hA coefficient (SEC_HA) | Htc*Area Coefficient of Secondary side fluid |
33,34 | Not used | |
35 | Mass or Volume (MASS_OR_VOL) |
Type of flow input for HOPT = 5 and 15 0. Flow in lbm/sec 1. Flow in gallon per minute |
36 | Overall HTC (HTC_OPT) |
Type of HTC input for HOPT=8 1. Fixed Overall HTC 2. Nusselt Number vs. Re Cold and Hot |
37 | Overall Heat Transfer Coefficient (OVERALL_HTC) | Overall Heat Transfer Coefficient Input |
38,39,43,44,45 | (NHT, NHT_1, NHT_2, NHT_3, NHT_4) | Table sizes |
40 | Select Mixed Flow (MIXED_FLOW) | 4. Mixed Flow side of HX for Cross flow one side mixed
option. 1=Primary, 2=Secondary |
41 | HX Area (HX_AREA) | The total heat exchanger area to be used with the Hs performance parameter |
42 | Hs Parameter (HS_PARAMETER) |
A Heat Exchanger Performance Parameter |
46 | NTU Constant (NTU) | The Number of transfer units for the “NTU Constant” heat transfer option. |
47 | Part of HX Matrix (MATRIX_MODE) | Indicates if this GHX is a standalone heat exchanger or is
being modeled as part of a matrix. 0) No 1) Yes, but not master. 2) Yes, and this GHX is the master. |
48 | Master GHX for Matrix (MTX_MASTER_ID) | The component ID of the GHX that is considered the master for the matrix. The master contains all the flow and heat transfer inputs. |
49 | Discretize Mode (DIS_OPTION) | The method used to take the overall heat transfer performance
information from the matrix master and apply it to all GHX
components in the matrix. 0) NTU scaling 1) Evenly distribute Q |
T1, T2, T3, T4 | Flow vs Delta P (COLD_DELTA-P, COLD_FLOW, HOT_DELTA-P, HOT_FLOW) |
|
T1, T2, T3, T4 | Fluid Velocity vs Delta P (COLD_DELTA-P,
COLD_VEL, HOT_DELTA-P, HOT_VEL) |
User-defined curve for Fluid Velocity vs. Delta.P. Delta-P table is the difference between the upstream driving total pressure and downstream sink static pressure. |
T1, T2, T3, T4 | Loss Coefficient vs Reynolds Number (COLD_KLOSS, COLD_REYN, HOT_ KLOSS, HOT_REYN) |
User-defined curve for Loss Coefficient vs. Reynolds Number. Reynolds Number= (4.0 * W/ (PERIM * μ)) Where:
If Hydraulic Diameter is provided, then:
If Hydraulic Diameter or Perimeter is not
Provided, Reynolds number is calculated based on Orifice
Area:
|
T5, T6, T7 | Effectiveness vs. Flow_Rate_Cold vs. Flow_Rate_Hot (FLOWRATE_COLD, FLOW_HOT.., EFFECTIVENESS) |
3D Table for Effectiveness vs. Flow_Rate_Cold vs Flow_Rate_Hot |
T5, T6, T7 | Effectiveness vs. NTU vs Heat Capacity Ratio (NTU, ..HEAT_RATIO, EFFECTIVENESS) |
3D Table for Effectiveness vs. NTU vs Heat Capacity Ratio |
T8, T9, T10 | Nusselt Number vs. RE_Cold vs. RE_Hot (NusseltNumber, ReynoldsCold, ReynoldsHot) |
3D Nusselt Number vs. RE_Cold vs RE_Hot |
T1, T2, T11 | Cold Flow vs. Delta P vs. T (COLD_DELTA-P, COLD_FLOW, COLD_FLOW_T) |
3D Table for Cold Flow vs. Delta P vs. T |
T3, T4, T12 | Hot Flow vs. Delta P vs. T (HOT_DELTA-P, HOT_FLOW, HOT_FLOW_T) |
3D Table for Hot Flow vs. Delta P vs. T |
Generic Heat Exchanger Theory Manual
Nomenclature: | |
---|---|
W : Mass flow rate | C: Heat Capacity |
ρ : Density | Q: Heat Addition/Rejection |
Cp : Specific Heat | Tt: Total Temperature |
NTU: Number of Transfer Units | |
Subscripts: | |
in, up, 1: Upstream station | C: Cold |
ex, dn, 2: Downstream station | H: Hot |
Pressure Loss Calculations
- 0. No Loss
- 1. Fixed Loss Coefficient
- 2. Fixed Total Pressure Drop
- 3. Flow vs Delta.P (PTIN – PSEX)
- 4. Velocity vs Delta.P (PTIN – PSEX)
- 5. Loss Coefficient vs Reynolds Number
- 6. Flow vs Delta P vs Delta T
As discussed above, Generic Heat Exchanger uses Orifice or Effective Area elements (Compressible or Incompressible) in the backend to model restrictions losses (pressure loss). The “No Loss” option uses a Link element. This can be the fastest run time option for GHX matrix models when pressure loss is not important.
- Fixed Loss Coefficient and Fixed Pressure Drop input refer to the Orifice Documentation.
- Curve based inputs (3-6) mentioned above refer to the Effective-Area Orifice Documentation
Heat Transfer Calculations
- Heat Input (Qin)
Tt,ex,Cold=Tt,in,Cold+QinWCold*Cpavg,Cold
Tt,ex,Hot=Tt,in,Hot−QinWHot*Cpavg,Hot
- Hot Fluid Delta.T
Q= WHot*Cpavg,Hot (Tt,in,Hot−Tt,ex,Hot)
Tt,ex,Cold=Tt,in,Cold+QWCold*Cpavg,Cold
- Cold Fluid Delta.T
Q= WCold*Cpavg,Coldt (Tt,ex,Cold−Tt,in,Cold)
Tt,ex,Hot=Tt,in,Hot−QWHot*Cpavg,Hot
- Effectiveness
CHot= ABS(WHot)*Cpavg,Hot
CCold= ABS(WCold)*Cpavg,Cold
Cmin=min(CHot, CCold)
QMax= Cmin*(Th,in−Tc,in)
Q= QMax*Effectiveness
Tt,ex,Cold=Tt,in,Cold+QWCold*Cpavg,Cold
Tt,ex,Hot=Tt,in,Hot−QWHot*Cpavg,Hot
- Effectiveness vs Flow_Rate_Cold vs
Flow_Rate_Hot
Effectiveness is obtained from User Defined Input for Effectiveness as function Flow_Rate_Cold and Flow_Rate_Hot.
CHot= ABS(WHot)*Cpavg,Hot
CCold= ABS(WCold)*Cpavg,Cold
Cmin=min(CHot, CCold)
QMax= Cmin*(Th,in−Tc,in)
Q= QMax*Effectiveness
Tt,ex,Cold=Tt,in,Cold+QWCold*Cpavg,Cold
Tt,ex,Hot=Tt,in,Hot−QWHot*Cpavg,Hot
- Effectiveness vs NTU vs Heat Capacity Ratio
Effectiveness is obtained from User Defined Input for Effectiveness as function NTU and Heat Capacity Ratio.
NTU= UACmin
CRatio= CminCmax
UA is calculated from Constant user input or from curve specified for Nusselt Number as function of Reynolds Number Cold and Reynolds Number Hot
UA= Nusselt_Number *KDh
Effectiveness=f(NTU, Cratio, Configuration)
CHot= ABS(WHot)*Cpavg,Hot
CCold= ABS(WCold)*Cpavg,Cold
Cmin=min(CHot, CCold)
QMax= Cmin*(Th,in−Tc,in)
Q= QMax*Effectiveness
Tt,ex,Cold=Tt,in,Cold+QWCold*Cpavg,Cold
Tt,ex,Hot=Tt,in,Hot−QWHot*Cpavg,Hot
- Nusselt Number vs RE_Cold vs RE_Hot
Nusselt Number is obtained from User Defined Input for Nusselt Number as function of Reynolds Number Cold and Reynolds Number Hot
UA= Nusselt_Number *KDh
NTU= UACmin
Effectiveness=f(NTU, Cratio, Configuration)
CHot= ABS(WHot)*Cpavg,Hot
CCold= ABS(WCold)*Cpavg,Cold
Cmin=min(CHot, CCold)
QMax= Cmin*(Th,in−Tc,in)
Q= QMax*Effectiveness
Tt,ex,Cold=Tt,in,Cold+QWCold*Cpavg,Cold
Tt,ex,Hot=Tt,in,Hot−QWHot*Cpavg,Hot
- Constant hA Coefficient value
CHot= ABS(WHot)*Cpavg,Hot
CCold= ABS(WCold)*Cpavg,Cold
Cmin=min(CHot, CCold)
1UA=(1h*A)Cold+(1h*A)Hot
NTU Effectiveness Methods:- Cross Flow Unmixed
Effectiveness=1−e((1CRatio)NTU0.22e(−CRatioNTU0.78−1))
- Counter Flow
Effectiveness= 1−e−NTU(1−CRatio)1−CRatioe−NTU(1−CRatio)
- Parallel Flow
Effectiveness= 1−e−NTU(1+CRatio)1+CRatio
- Cross Flow Both Side Mixed
Effectiveness= 11(1−e_NTU)+CRatio1−e−CRatioNTU−1NTU
- Cross Flow One Side Mixed
Cmin is mixed: Effectiveness=1−e−(1CRatio)(1−e−CRatioNTU)
Cmax is mixed: Effectiveness= (1CRatio)(1−e−(CRatio(1−e_NTU)))
QMax= Cmin*(Th,in−Tc,in)
Q= QMax*Effectiveness
Tt,ex,Cold=Tt,in,Cold+QWCold*Cpavg,Cold
Tt,ex,Hot=Tt,in,Hot−QWHot*Cpavg,Hot
- Cross Flow Unmixed
- Hs Parameter Methods (Constant and vs Hot and Cold
Flowrates)
The Hs parameter is typically used to describe radiators where the hot side is a liquid coolant, and the cold side is air.
Hs=ABS(WHot)*Cpavg,Hot*(Th,in−Th,ex)HXArea*(Th,in−Tc,in)
Hs*HXArea*(Th,in−Tc,in)=ABS(WHot)*Cpavg,Hot*(Th,in−Th,ex)=Q
A heat exchanger effectiveness can be calculated using the Hs parameter.
Effectiveness=Q / QMax
QMax= Cmin*(Th,in−Tc,in)
Effectiveness=Hs*HXArea*(Th,in−Tc,in)Cmin*(Th,in−Tc,in)=Hs*HXAreaCmin
Q is found using the effectiveness Qmax. The Q is applied to the fluid streams to get the exit temperatures.
Tt,ex,Cold=Tt,in,Cold+QWCold*Cpavg,Cold
Tt,ex,Hot=Tt,in,Hot−QWHot*Cpavg,Hot
- Fixed Hot (or Cold) Fluid Exit Temperature
Specify the temperature at the exit of the heat exchanger for the hot or cold stream. The heat flow (Q) is calculated for a hot (or cold) stream and applied to both streams.
Q= WHot*Cpavg,Hot (Tt,in,Hot−Tt,ex,Hot) or Q= WCold*Cpavg,Cold (Tt,in,Cold−Tt,ex,cold)
Tt,ex,Hot or Tt,ex,Cold are specificed as inputs.
- Hot (or Cold) Fluid Exit Quality
Specify the fluid quality at the exit of the heat exchanger for the hot or cold stream. The heat flow (Q) is calculated for a hot (or cold) stream and applied to both streams. The solver calculates the Q from the enthalpy change required to change the quality to the target exit value. The source of the fluid properties must be Coolprop for this option. Also, the energy balance option must be “enthalpy based energy balance”. The energy balance option is found in
. - NTU Constant
Specify the NTU for the heat exchanger. The NTU, hat capacity ratio, and HX configuration are used to calculate the heat flow (Q).
Effectiveness=f(NTU, Cratio, Configuration)
Qmax=Cmin*(Th,in−Tc,in)
Q=QMax*Effectiveness
Generic Heat Exchanger Matrix
- NTU scaling.NTUlocal GHX= NTUfullNumber of GHX in matrix*Cmin,totalCmin,local
- Evenly distribute Q.
Q is calculated for the total HX and distributed evenly to each.
Generic Heat Exchanger Outputs
The following listing provides details about Generic Heat Exchanger Component output variables.
Name | Description | Units |
---|---|---|
PS | Static pressure | psia, MPa |
PT | Total pressure | psia, MPa |
TT | Total temperature of fluid | deg F, deg K |
RE | Reynold Number | (None) |
Rho | Density | lbm/ft^3, Kg/m^3 |
CP | Specific Heat | Btu/(Lbm R), kJ/Kg.K |
K | Thermal Conductivity | Btu/(hr ft R), W/m.K |
DVISC | Dynamic Viscosity | Lbm/(hr ft), N s/m^2 |
Area | Flow Area | In2, m2 |
Heat Mode | Heat Mode Options (An echo of the user input). | (None) |
Pri/cold_side_ploss_opt | Options specified for Pressure Loss Modeling for Pri/Cold side. (An echo of the user input). | (None) |
Sec/hot_side_ploss_opt | Options specified for Pressure Loss Modeling for Sec/Hot side. (An echo of the user input). | (None) |
Q | Heat Transferred between Heat Exchanger fluids | BTU/sec, W |
Effectiveness | Effectiveness of Heat Exchanger | (None) |
Pri/cold_side_delta.p | Delta.PT on Pri/Cold side flow | psia, MPa |
Sec/hot_side_delta.p | Delta.PT on Sec/Hot side flow | psia, MPa |
Mdot | Mass Flow Rate | Lbm/s, kg/s |
Flow configuration | Heat Exchangers Flow Configurations. (An echo of the user input). | (None) |
Overall conductance(UA) | Overall Thermal Resistance | BTU/hr.F, W/K |
NTU | No of Transfer Units | (None) |
Pri_hA_out | Pri/Cold side flow hA Coefficient value | BTU/hr.F, W/K |
Sec_hA_out | Sec/Hot side flow hA Coefficient value | BTU/hr.F, W/K |
Virtual Element | The element number created at run time for the two flow streams. The virtual element calculates the flow and pressure loss information so that it can be viewed in the .res file for additional details. | (None) |
Heat Capacity | Mass flow rate x Fluid Specific Heat | BTU/sec/degF, W/degK |
Heat Capacity Ratio | The minimum heat capacity over the maximum heat capacity. | (None) |